Articles
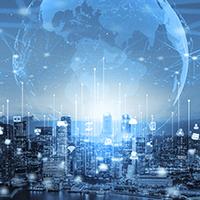
Ubiquity
Volume 2023, Number August (2023), Pages 1-15
Digital Economy: Electricity Consumption by ICT: Facts, trends, and measurements
Erol Gelenbe
DOI: 10.1145/3613207
This paper considers key issues surrounding energy consumption by information and communication technologies (ICT), which has been steadily growing and is now attaining approximately 10% of the worldwide electricity consumption with a significant impact on greenhouse gas emissions. The perimeter of ICT systems is discussed, and the role of the subsystems that compose ICT is considered. Data from recent years is used to understand how each of these sub-systems contribute to ICT's energy consumption. The quantitatively demonstrated positive correlation between the penetration of ICT in the world's different economies and the same economies' contributions to undesirable greenhouse gas emissions is also discussed. The paper also examines how emerging technologies such as 5G, AI, edge computing, and cryptocurrencies are contributing to the worldwide increase in electricity consumption by ICT, despite the increases in ICT efficiency, in terms of energy consumed per bit processed, stored, or transmitted. The measurement of specific ICT systems' electricity consumption is also addressed, and the manner in which this consumption can be minimized in a specific edge computing context is discussed.
Energy consumption by ICT and its CO2 impact are controversial subjects where experts of different organizations and tendencies may express diverse and sometimes divergent views [1, 2, 3, 4, 5, 6, 7, 8, 9, 10, 11]. One reason for some of these controversies is the lack of precise definitions regarding the perimeter that defines "ICT systems," for instance should home entertainment, the technologies that are now part of the entertainment industries, or the financial fintech industry, fully enter into the picture. The relative lack of systematic measurement data about the energy consumption of digital devices and systems is yet another source of uncertainty and controversy.
Figure 1 is a simplified description of the broad perimeter of ICT systems, describing the type of hardware and systems that are deployed, while end users typically see only their own devices (hardware) and the numerous applications (software) that they use. Yet ICT's energy consumption, and the resulting CO2 generated, are a consequence of the hardware that is used and the manufacture of that same hardware. Indeed, ICT manufacturing consumes a major amount of electricity, and also results in the consumption of other forms of energy for the extraction of minerals needed to manufacture ICT components and systems.
The radio access network (RAN) transitioned over many decades through various generations of hardware and standards (1G, 2G, 3G, 4G, LTE), and is now moving toward 5G; in the future it may become 6G. The RAN uses wireless to connect to mobile phones and other devices such as body sensors, as well as parts of the Internet of Things (IoT) including road traffic monitoring, smart homes, the smart grid, smart cars, some of the industrial IoT or "Industrie 4.0", and other applications. It is connected by wire, optical fiber, and wireless (including terrestrial and space-based wireless), through the mobile operator's backhaul networks, to the mobile core network which is mainly fiber and wire, with routers and switches that connect to edge systems, data centers, and the cloud. The RAN also uses fog and edge devices in close physical proximity to wireless base stations of the RAN, that offer access with low latency to data and other end users for interactive or data and video intensive games, entertainment, or the IoT, and then connects to the internet composed of routers, switches with mostly fiber connectivity.
The internet interconnects powerful data centers, billions of homes with entertainment boxes, Wi-Fi routers, businesses, buildings with high speed wired or fiber local area networks (LAN), as well as the IoT for control applications, e.g., industrial, heating and cooling, home and building security, vehicle charging stations, publicly available bicycles, electric vehicles and their charging stations, and public transport.
The Challenge of Measuring ICT's Electricity Consumption and CO2 Impact
Although it may be technically possible to measure the electricity consumed by ICT globally, this may well be practically infeasible [8, 9, 10], since it would require that industry and business measure their energy consumption:
- to manufacture, sell or deliver ICT hardware,
- to produce software,
- and when they operate ICT systems.
Monitoring of energy consumption for the operation and cooling of ICT equipment is often carried out by data center and communication network operators, though it is obviously hard to do for many billions of devices that are manufactured and used. It would also require the means to store, analyze, and disseminate this data over long periods of time which in itself would use much storage, transmission, and processing capacity, and cause additional energy consumption.
As an example of a specific particular device, the measured power and energy consumption characteristics of a widely used Intel NUC processor for edge systems [12] is shown in Figure 2.
It is also difficult to estimate the fraction of electricity consumed to secure ICT devices and services, and to defend them from cyberattacks. One effect of cyberattacks that is actually desired by attackers, is to increase the operating costs of the "victim," and one way of achieving this is to increase the electricity consumed in computer systems networks when they are attacked. This is caused by the added traffic, plus the electricity consumed within systems whose workload and processing may also increase during an attack or even during normal operation, in order to deter, counter or mitigate attacks [13].
Worldwide Estimate of Electricity Consumption by ICT and its CO2 Impact
Turning now to overall electricity consumption figures for ICT, the International Energy Agency (IEA) provided the following estimates for the year 2019, shown in Table 1, which were updated for 2023 [3].
Since 2020 and 2021 were "untypical" years due to Covid, it would be best to let the year 2022 go by, and then examine the figures for 2022 to see whether the growth both of electricity consumption and of CO2 impact of ICT has been recently increasing at the same 2–3% annual irate as in the past decade.
Indeed, ICT has substantially increased its overall share of, going from 4–5% a decade ago, to currently 8–10% of total electricity production. The overall share of carbon emissions reported varies between close to 2% and up to 4%, comparable but probably higher to the CO2 impact of air travel as a whole [2, 3]. This growth is illustrated in a 2019 research brief from Ericsson [14] that covers ICT network operations in 2010 and 2015, which is summarized in Figure 3.
Certain ICT industry sectors privilege the purchase of energy from renewable sources to improve their CO2 emissions. Although this encourages electric power companies to increase their renewable energy supplies, it can also encourage the production or transfer of non-renewable energy sources to other sectors of the economy (or to neighboring countries). An example is the case of Canada, which produces nearly 60% of its electricity from renewable sources (such as hydroelectricity) and uses some of it to extract the shale gas and oil that it exports. Of course, carbon emissions per KWh of electricity vary widely from one country to another based on the primary sources of energy that are used; countries such as Belgium and France, which generate most of their electricity via nuclear plants, have a very low average CO2 emission well under 100g per KWh of electricity. Another environmental concern regarding ICT that is seldom mentioned, is that digital chips currently use nearly two-thirds of the elements of the periodic table, many of which require substantial energy expenditure for their extraction. Additionally, the materials used within ICT systems can be significant polluters when ICT equipment is being decommissioned.
As a rough estimate, if we assume 10% of any ICT equipment is routinely consumed for cybersecurity and 20% of ICT system operations relate to dealing with access control and cryptography, virtual private networks, system patches, security updates and upgrades, and blockchain—which may be increasingly used for applications that require certification—plus dealing with attacks themselves, we then arrive at an energy cost of 300 TWh annually devoted to cybersecurity. At an average of approximately 485g of CO2 per KWh of electricity that is produced worldwide (this is a recent figure from the IEA), it results in a rough estimate of 145.5 million metric tons of CO2. However, if we consider most operations used for online banking and payments are related to authentication and security, it is likely we would reach significantly higher estimates of the amount of electricity that is actually used for securing our ICT systems.
Can High ICT Penetration Reduce Carbon Emissions
Obviously, experts from the computer industry will praise the ever-increasing efficiency of digital equipment, although this is typically done in terms of energy consumption per volume of data that is processed, stored, or transmitted. However, experts who are most concerned with climate change and emissions, tend to stress ICT's constant increase in energy consumption by ICT, and the presence of rare and polluting materials that are used to manufacture semiconductors and digital systems.
While ICT is often presented as a means or energy savings and reduction of CO2 emissions in various areas, there appears to be little overall evidence to this effect, since current data suggests those countries that have some of the world's highest CO2 emissions are often the ones with the highest penetration of ICT as a share of their economies. This effect has been studied by some authors [15], and is shown in Table 2; the "Bech Index" or share of business-to-business economic activity in ICT taken per country seems to be highest in many countries where energy consumption and overall CO2 impact is high. This should not be surprising, since recent economic development has gone hand in hand with high energy consumption as well as a high degree of automation in all sectors of activity.
Some countries that have moved away from manufacturing toward tertiary and service-based activities, appear to have maintained a relatively stable or slightly decreased consumption of electricity and primary energy, while they have also experienced a significant increase in "imported CO2" due to transport and manufacturing of their imported goods from abroad. While internet-based work from home and home schooling during the Covid-19 pandemic have reduced the energy consumed for transportation and for running large buildings and campuses, home-based activities may have also increased the energy consumption for heating, cooking, or cooling at home. The analysis of ICT usage, energy consumption and overall CO2 impact during Covid-19 could enlighten some of these aspects.
However, as a whole, and on a country-by-country and worldwide aggregate basis, there is no hard evidence to date that would suggest the increased penetration of ICT has actually reduced overall energy or electricity consumption and CO2 impact. On the other hand, we see some studies regarding China are investigating the links between economic development, ICT penetration in the economy, and carbon emissions [16]. Since China is a large country with significant regional differences, such studies can provide insight into this important matter, provided they include the effect of "imported carbon emissions," resulting from goods consumed or used locally, but manufactured elsewhere and imported from other cities or regions.
The Effect of Evolving Technologies
ICT research and industry have constantly, since their origins in the 1940s, pursued and achieved higher levels of performance, greater processing speeds, and faster data transmission rates. These advances have been accompanied by a constant increase in the penetration of ICT into all sectors of society and the economy, and offered great gains in research, healthcare, audio and visual fidelity in entertainment, the expansion of e-commerce and the e-economy, and other sectors. However, this has also come with a steady increase in energy consumption and CO2 impact related to ICT.
In the current context in 2023, we are transitioning through two such technological evolutions, toward full deployment of 5G regarding mobile networks, and toward the greater use of fog and edge computing to complement the cloud and data centers that support mobile networks. These transitions are good examples about the manner in which ICT energy consumption also evolves.
GSMA, the organization that represents mobile operators and the telecommunication industry worldwide, has estimated that currently 20–40% of the operating cost of network operators is taken up by electricity, and that 5G can cause a substantial (as much as four to five fold) increase of energy consumption in the RAN, but that the technical means to reduce this consumption may be included in later generation mobile networks [4]. Together with the RAN, fog and edge devices that offer low latency access to data for mobile applications—such as the IoT, online games, or smart vehicles by bringing large files to the physical proximity of mobile base stations of the RAN—cannot replace the need for permanent data storage in the cloud, but may reduce the need for large data centers.
Recently, ICT electricity consumption has also increased through the use of cryptocurrencies (both "mining" and sales), and "blockchain technology" and distributed ledgers for securing contractual agreements, which require a large number of concurrent distributed transactions, have consumed [5 , 17] some 120 TWh of electricity per year, exceeding the electricity consumption of the Netherlands. Yet another usage of computing that can consume significant electricity is machine learning, which may also be used to optimize certain other processes and reduce energy consumption in other areas [18].
A way forward to reduce energy consumption by ICT has been suggested by our research in the recent European Union H2020 projects SerIoT and IoTAC [12, 19], where reinforcement learning was shown to reduce energy consumption in an edge system at the cost of increasing average system response time to jobs by a few percent, with a significant reduction of power consumption in edge systems of the order of 10–15% , as shown in Figure 4.
Energy consumed for the manufacturing of ICT equipment may be reduced and optimized by improving and upgrading ICT systems to gradually improve system performance without changing all the equipment, by recycling existing equipment for other uses, and optimizing the instants when a system is replaced so as to maximize performance while minimizing major energy consumption costs linked to equipment manufacturing and delivery.
Conclusions
While new technologies, such as quantum computing, may someday affect the continued increase of the amount of electricity consumed by ICT, and the offsets that it can create in greenhouse gas emissions in other areas and industries, ICT today is still reliant on the silicon-based semiconductor industry and conventional digital systems. We observe that the increased use of new booming technologies such as blockchain and AI [17, 18], as well as the deployment of 5G networks, are fueling the continued increase in energy consumption by ICT, while corporate data centers and networks are increasing their use of renewable energy sources.
Thus, this paper has focused on the electricity consumption of ICT, with the understanding that its own direct impact on greenhouse gas emissions will depend significantly on the sustainability of electricity production itself. On the other hand, we have stressed the importance of limiting and optimally reducing the growth of electricity consumption by ICT by managing our ICT systems judiciously, reusing or improving existing systems, and replacing them in a manner that also considers the resulting overall energy consumption, together with the quest for better performance in communications and computing. Broader considerations regarding many aspects of industry, agriculture, and energy, including ICT, are addressed in a report from the International Council of Academies of Engineering and Technological Sciences [20].
Much work remains to be done in this broad field, and one thing that is certain is that we need to understand and optimize the multiple links between computer science, ICT, and energy, so as to be better armed to face the challenges of climate change. Thus, a way forward that may be addressed by computer scientists is to develop methods that analyze the interaction between energy consumption, computation, and communication, such as the "energy packet network paradigm" [21, 22, 23].
Where does the competitive exclusion principle fit in? Coinbase Global, a company that makes a market in cryptocurrencies, is the leading cryptocurrency exchange platform and is rapidly becoming the dominant exchange with 56 million users and 11% market share [8]. While it may not dominate forever, Coinbase is leveraging its advantage—just as Gause's competitive exclusion principle predicts. But the market cap of all cryptocurrencies is by far less than fiat currencies. It remains to be seen if they will benefit from the exclusion principle. So far, they have not.
Acknowledgements
The author gratefully acknowledges the support of several EU Research Programs in forming the opinions and observations expressed in this paper, including FP7 Fit4Green, ECROPS, FP7 NEMESYS, H2020 SDK4ED, SerIoT and IoTAC. He has also benefited from discussions in several working groups, including the CAETS Energy Working Group, while he was drafting its chapter on ICT and Energy [22]. The feedback he received after giving keynotes in recent years at the French Ministry of Economics ICT Energy Conference, the Turkish Academy of Sciences Energy Committee, and the IEEE ISTAS Symposium 2022 [10], are also acknowledged.
References
[1] Ferreboeuf, H. et al. Lean ICT: Towards digital sobriety. The Shift Project. March 2019; https://theshiftproject.org/en/article/lean-ict-our-new-report.
[2] Gelenbe, E. and Caseau, Y. The impact of information technology on energy consumption and carbon emissions. ACM Ubiquity 15, June (2015) https://doi.org/10.1145/2755977.
[3] IEA. Data centers and data transmission networks. IEA, Paris. July 11, 2023; https://www.iea.org/energy-system/buildings/data-centres-and-data-transmission-networks#overview.
[4] GSMA. Energy efficiency: An overview. 2019.
[5] ACM TechBrief: Computing and Climate Change, ACM Technology Policy Council (Issue 1, November 2021).
[6] Fagas, G., Gallagher, J. P., Gammaitoni, L. and Paul, J. P. Energy challenges for ICT. In ICT - Energy Concepts for Energy Efficiency and Sustainability. IntechOpen. 2017.
[7] Masanet, E., Shehabi, A., Lei, N., Smith, S., and Koomey, J. Recalibrating global data center energy-use estimates. Science 367, 6481 (2020), 984–986.
[8] Pernici, B. et al. What IS can do for environmental sustainability: A report from CAiSE'11 Panel on Green and Sustainable IS. Communications of the Association for Information Systems 30, 1 (2012).
[9] Enerdata. Electricity domestic consumption. World Energy & Climate Statistics - Yearbook 2023.
[10] Gelenbe, E. Energy consumption by ICT: Facts and trends. Keynote paper. IEEE International Symposium on Technology and Society 2022 (ISTAS22). Virtual conference.
[11] Boiardi, S., Capone, A., and Sansò, B. Planning for energy-aware wireless networks. IEEE Communications Magazine 52, 2 (2014), 156–162. DOI:10.1109/MCOM.2014.6736757.
[12] Fröhlich, P., Gelenbe, E., Fiołka, J., Checinski, J., Nowak, M., and Filus, Z. Smart SDN management of fog services to optimize QoS and energy. Sensors 21, 9 (2021).
[13] Abdelrahman, O. H. and Gelenbe, E. Signalling storms in 3G mobile networks. 2014 IEEE International Conference on Communications (ICC). IEEE, 2014, 1017–1022. DOI: 10.1109/ICC.2014.6883453.
[14] Ericsson. Global electricity usage of ICT network operators — an extensive data set. Research paper. 2019.
[15] Bech, H. P. And the winners remain China and India. The Bech Index 2020. TBK Publishing. May 2020. DOI: 10.13140/RG.2.2.10093.41440.
[16] Zhao, X. and Zhang, X. Research on the evaluation and regional differences in carbon emissions efficiency of cultural and related manufacturing industries in China's Yangtze River basin. Sustainability 14, 17 (2022).
[17] As interest in cryptocurrencies and NFTs continues to grow, so too does the discussion around its energy consumption. But just how big is Bitcoin's energy bill? MoneySuperMarket.com. Sept. 16, 2022.
[18] Strubell, E., Ganesh, A., and McCallum, A. Energy and policy considerations for deep learning in NLP. In Proceedings of the 57th Annual Meeting of the Association for Computational Linguistics, Florence, Italy. Association for Computational Linguistics, 2019, 3645–3650. DOI: 10.18653/v1/P19-1355.
[19] Gelenbe, E., Domanska, J., Fröhlich, P. , Nowak, M. P., and Nowak, S. Self-aware networks that optimize security, QoS, and energy. Proceedings of the IEEE 108, 7 (2020), 1150–1167. DOI: 10.1109/JPROC.2020.2992559.
[20] International Council of Academies of Engineering and Technological Sciences (CAETS). Towards low-GHG emissions from energy use in selected sectors. CAETS 2022 Energy report. Feb. 27, 2023.
[21] Gelenbe, E. Energy packet networks: ICT based energy allocation and storage. In Rodrigues, J. J. P. C., Zhou, L., Chen, M., and Kailas, A. (eds.) Green Communications and Networking. GreeNets 2011. Lecture Notes of the Institute for Computer Sciences, Social Informatics and Telecommunications Engineering, vol 51. Springer, Berlin, Heidelberg, 2012.
[22] Gelenbe, E. and Ceran, E. T. Energy packet networks with energy harvesting. IEEE Access vol. 4 (2018), 1321–1331.
[23] Gelenbe, E. G-networks with instantaneous customer movement. Journal of Applied Probability 30, 3, (1993), 748.
Author
Erol Gelenbe FACM FIEEE FIFIP is Professor in the Institute of Theoretical and Applied Informatics of the Polish Academy of Sciences; he is also a researcher in the University Cote d'Azur, CNRS, I3S, 0600 Nice, France. His career has included chaired professorships at Liege University (Belgium), Paris-Saclay University, Paris-Descartes University, Duke University, and UCF and the "Dennis Gabor" Professorship at Imperial College. He served as Director of the Ecole des Hautes Etudes en Informatique at Paris-Descartes University (1986–1992), Chair of the ECE Dept at Duke (1993–1998), Director of the School of Electrical Engineering and Computer Science at UCF (1998–2003), and Head of Intelligent Systems and Networks at Imperial College London (2003–2018). He invented the eponymous computer and network performance models known as G-Networks which offer analytical solutions for systems that include adaptive control algorithms for task scheduling and routing, as well as the Random Neural Network and its learning algorithms. Based on this work he has developed patented tumor identification algorithms from human brain MRI images, patented adaptive routing algorithms for the Internet, adaptive video compression schemes for video-conferencing, and Intrusion Detection Systems for the Internet. His current research focuses on self-aware systems that optimize cybersecurity, quality of service and energy consumption, with support from the EU Horizon 2020 Program. He has also developed computational models for gene regulatory networks. Currently chair of the Informatics Section of Academia Europaea, he is a Fellow of the French National Academy of Technologies, the Belgian Royal Academy of Sciences, Arts and Letters, Academia Europaea, Polish and Turkish Science Academy, and Hon. Fellow of the Hungarian Academy of Sciences and the Islamic Acad. of Sciences. He has served as editor-in-chief of the Computer Journal (BCS) and SN Computer Science, and on the editorial board of the Proc. Royal Society A, IEEE Trans. of Software Engineering, and is currently on the editorial board of ACM Ubiquity, Acta Informatica, Performance Evaluation, and IEEE Trans. on Cloud Computing. He also has graduated some 95 Ph.Ds. He was awarded Knight of the Legion of Honour (France), Commander of Merit of France and Italy, Commander of the Crown of Belgium, and Grand Officer of the Star of Italy.
Figures
Figure 1. Simplified and schematic representation of ICT systems worldwide, describing the types of subsystems that are currently deployed.
Figure 2. The curve on the left shows the power consumption of a NUC, used as a packet router, versus its overall throughput in Mb/sec, the curve on the right shows its energy consumption per Mb as a function of the throughput (from the author's paper [12]).
Figure 3. The growth of electricity consumption in ICT network operations between 2010 and 2015, contained in a report by the Ericsson company [14].
Figure 4. The two graphs from recent work [12], show the effect of using a reinforcement learning based "real-time" policy to manage the allocation of tasks to servers of an edge platform, so as to minimizes a cost function that combines QoS and energy consumption, rather than just focusing on optimizing QoS. The graph on the right shows that the smart policy reduces power consumption by 10% (at high throughput) to 15% at low throughput), while the graph on the left shows that the response time increases by a tiny 2% to 3%, even at high throughput.
Tables
Table 1. Summary of the International Energy Agency's (IEA) estimates for the year 2019 of the electricity consumption (in terawatt-hours) worldwide by different sectors of ICT, namely data centers, networks including the radio access network and end users, and the manufacturing of ICT equipment, which represents roughly 50% of the total amount.
Table 2. Top ICT based economies (left) , based on the share of ICT in their GDP, and the list of countries and regional groups with highest CO2 emissions worldwide [15].
Copyright 2023 held by Owner/Author.
The Digital Library is published by the Association for Computing Machinery. Copyright © 2023 ACM, Inc.
COMMENTS